For a net-zero future
The B&W Learning Center
Leading the world in clean power production technology
Boiler Cycling
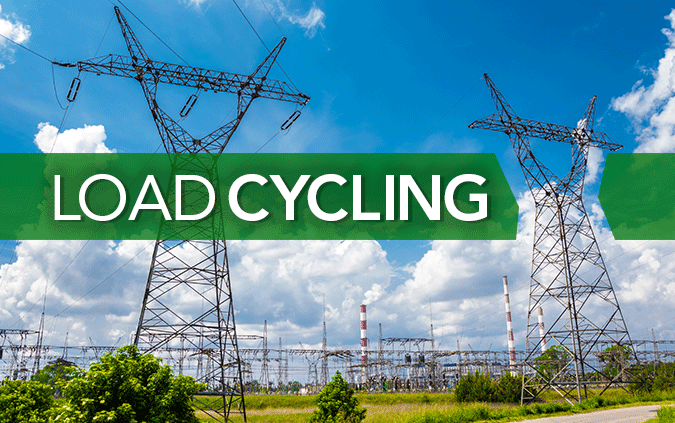
Boiler Cycling Considerations
Many fossil fuel-fired boilers are now experiencing load cycling or on/off cycling operation. These modes of operation involve cycling a unit on and off line or reducing loads to absolute minimums in response to varying load demand. This can be caused by daily or weekly load shifts experienced on nights and weekends, power generators dispatching units to the grid which utilize a lower cost fuel, such as natural gas, or the availability of alternate and less consistent generation sources such as solar or wind power.
Most of these units were originally designed for base loaded operation and not designed to accommodate frequent on/off cycles. The stresses placed on the weld joints, headers, drums and attachments between expanding and contracting parts, can cause failures after a finite number of cycles. Cycling operation can shorten the life span of such equipment based on number and duration of cycles. A thorough condition assessment program can determine potential equipment modifications that can make the unit more flexible and alleviate possible problem areas. Technology developments for the newest units designed for cycling have made a wide variety of steam generator modifications available to be retrofitted to baseload designs.
Cycling Effects and Solutions
In assessing a boiler’s ability to withstand cycling, those components most vulnerable to cycling are reviewed first. These components are discussed from two standpoints: the operating methods which minimize cyclic damage, and design modifications which permit the component to better withstand cycling conditions.
Cycle definition
Two types of cycling service are usually considered: load cycling and on/off cycling.
A load cycle is considered to start at full load, full temperature steady-state conditions. It goes through a load reduction, then returns to the initial conditions. A typical load cycle is composed of three phases:
- load reduction,
- low load operation, and
- reload.
A typical on/off cycle has four phases:
- load reduction,
- removal from operation
- restart, and
- reload.
The offline period can offer one area of potential for reducing cyclic damage. The following are issues related mainly to on/off cycling.
Economizer thermal shock
On boilers that are on/off cycled, economizers often show more cyclic damage than other components. The economizer receives water from the extraction feedwater heater system, and the inner metal surface temperature follows the feedwater temperature with practically no time delay. As a result, high rates of metal temperature change can occur with high local stresses.
Fig. 1 shows economizer inlet temperatures during an overnight shutdown cycle. The first two hours are for load reduction, followed by eight hours of idle condition. Next, the boiler is fired in preparation for restart. The rates of temperature change during the load decrease and increase are usually not excessive, but would represent load cycling conditions for the economizer.
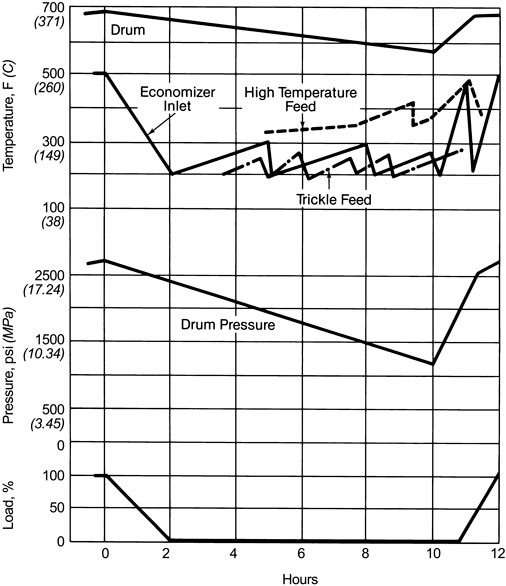
Fig. 1 Economizer temperatures during overnight shutdown cycles.
During the offline period, there is typically some air leakage through the boiler with a resulting decay in boiler pressure. As this happens, the drum water level decreases. At the same time, the air passing through the boiler is heated to near saturation temperature, and that air then heats the economizer. An economizer metal temperature can increase at 30 to 50F/h (17 to 28C/h) during this period and can approach saturation temperatures. When the drum level drops as the unit cools, the operator usually reestablishes drum level by adding feedwater so the unit is ready for firing. Because there is no extraction steam available, the feedwater temperature is low. This slug of cold water quickly cools the economizer, causing thermal shock as indicated by the solid lines in Fig. 1. Typically, the inlet header and inlet tubes receive the greatest shock.
When the boiler is fired in preparation for turbine restart, rollup and synchronization, the economizer heats up rapidly, often nearing saturation temperature. Feedwater flow is started when the initial load is applied to the turbine. Because little extraction heating is available, feedwater temperature is initially low. A thermal shock can occur as extractions are cut into feedwater heaters and the temperature can increase several hundred degrees F (C) in a few minutes.
Typically, early damage consists of cracks initiating in the tube bore holes of the inlet header which are closest to the feedwater inlet connection.
Other damage has also been seen from this cyclic service. Outlet headers have shown damage similar to inlet headers. Furthermore, some tube bank support systems cannot accommodate the high temperature differences between rows.
Solutions are available to reduce the frequency and magnitude of thermal shocks. These address the out-of-service and restart conditions. One solution is called trickle feed cooling. Very small quantities of feedwater are frequently introduced during the shutdown and restart periods. This prevents the inlet header from heating and reduces the cooling rate during feedwater introduction. Because feedwater introduction is controlled to limit economizer temperature rise, some drum blowdown may be necessary to prevent a high water level.
A second method of reducing thermal shock is to permit the economizer to reheat during the idle period and then to provide higher temperature feedwater for restart. This can occur by pressurizing a high-pressure heater with steam from an auxiliary source or from the drum of the unit. The quantity of steam required is low because it only heats the initial low flow of feedwater.
Furnace subcooling
Drum boilers that have been subjected to on/off cycling have also been found to develop multiple cracks in lower furnace wall tubes where the tubes are restrained from expansion or contraction. Typical cracking areas have been at the lower windbox attachment where filler bars or plates are welded to the tubes. (See Fig. 2.)
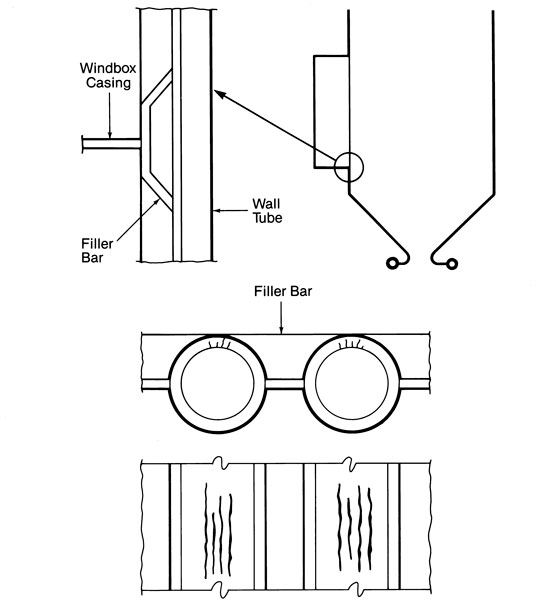
Fig. 2 Lower windbox attachment cracking due to subcooling.
Investigation of these failures indicated that, during the shutdown or idle period, relatively cold (cooled below saturation temperature) water settled in the lowest circuits of the furnace bottom. When circulation was started by initial firing or the circulating pump, the cold-water interface is moved upward through the walls, rapidly cooling the tubes. As the cooler water moves up the furnace its temperature gradient is decreased and as such the rate of cooling is decreased, therefore reducing damage higher in the furnace. Experience indicates that if this subcooling can be limited to 100F (56C), there is a low probability of damage.
An out-of-service circulating pump system may be used to limit the subcooling. (See Fig. 3.) This is a low capacity pump that draws from the bottom of the downcomers and discharges water to the drum, therefore preventing the stratification temperatures of water within the unit.
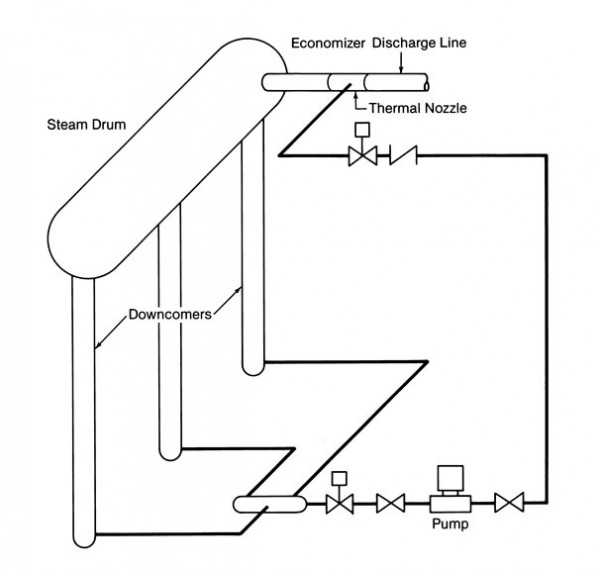
Fig. 3 Out-of-service circulating pump system.
Corrosion fatigue
The impact of on/off cycling continues to present itself to other areas of the boiler as the unit ages and the number of cycles increases. Circuits such as supply tubes and risers are susceptible to damage given enough years in operation and enough cycles. Owners must be particularly alert to damage in circuits that are outside the boiler enclosure and pose greater safety hazards in the event of failure. B&W has prepared a plant service bulletin discussing this topic.
Tube leg flexibility
The enclosure walls of most boilers are water- or steam-cooled. The water-cooled circuits carry boiling water, and the steam-cooled circuits carry steam from the drum. As a result, they operate near the saturation temperature corresponding to the drum pressure. Whether the boiler is being fired or shut down, considerable heat absorption or loss is necessary to change the temperature of the walls. As a result, they change temperature more slowly than the other components.
The economizer, superheater and reheaters penetrate these walls; the penetrations are designed to be gas-tight. At the point of penetration, the side-to-side expansion follows saturation temperature. However, the header that forms the inlet or outlet of the component expands with the temperature of the steam or water that it contains. Fig. 4 shows the typical motions of the header end and the outermost connecting leg for a superheater or reheater outlet. Note that the greatest deflection is when the header temperature is at a maximum.
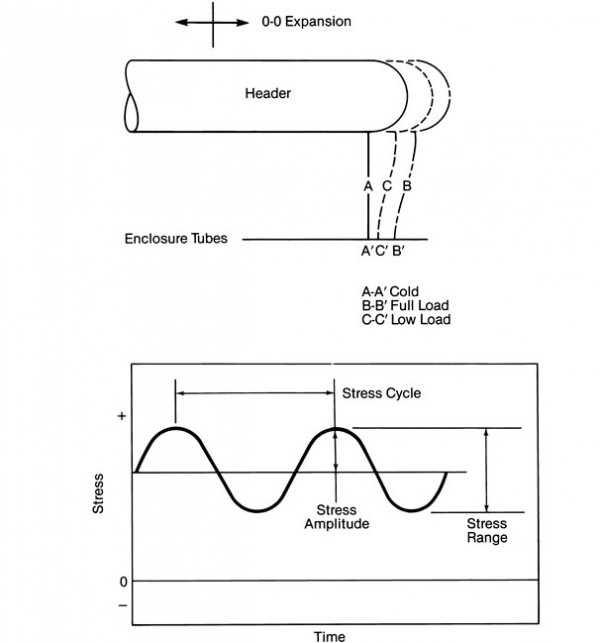
Fig. 4 Superheater tube leg flexibility.
In the case of economizers and the reheater inlet, the deflection is in the opposite direction because these headers operate near or below saturation temperature. For these components the greatest temperature difference, and therefore the greatest deflection, is at low loads.
Regardless of the direction, the greatest temperature difference produces the maximum differential expansion and the maximum bending stresses in the connecting legs. The stress range and amplitude dictate component fatigue life.
The first indications of cyclic damage are external cracks on the tube-to-header welds or the stub-to-tube welds of the outermost header legs. This damage is relatively easy to inspect and repair. Successive damage is also usually limited to closely adjacent legs because they have experienced similar stress levels. Most sensitive are high-temperature headers located at relatively short distances from the penetration seal on large units. On large boilers, the headers are long which, when coupled with short outlet tube legs, will produce the greatest bending stresses at the header welds.
Steam drums
The cycling of utility drum boilers has the potential to produce significant variation in drum temperatures. The drum is a large thick-walled vessel that inherently responds slowly to temperature changes. Top-to-bottom variations in drum temperature can lead to drum humping, excessive stresses on connections, and fatigue in welds.
Any areas of significant temperature variations, including nozzle penetrations, have the potential to produce excessive thermal stresses and drum damage over time, given enough cycles. Consequently, the drum must be addressed when cycling operation is to be the norm.
The steam drum should be included in a condition assessment program to identify evidence of any damage that has occurred. However, operating conditions that can lead to damage should be mitigated. Operating data should be analyzed or instrumentation installed to assess drum upsets. Thermocouples can be installed on the drum to monitor temperature differentials. A review of the boiler operating history can verify that the rate of pressure (saturation temperature) changes are controlled. If cycling upsets cannot be controlled by changes to operating practices, system upgrades may be necessary.
Cycling Boiler Circulation Upgrade
As previously mentioned, furnace subcooling, boiler-forced cooling during a shutdown, and intermittent cold feedwater flow into the boiler during startup are three sources of thermal differentials and cyclic cracking. The thermal stresses produced within the components may be sufficient to produce low cycle fatigue cracks. The solution in most cases is to modify the boiler and/or feedwater system to prevent the sudden entry of cold water into hot boiler components.
When a boiler is experiencing cracking due to subcooling and cold feedwater flow upon startup, one solution is to install an offline pump-assisted circulation system to reduce the thermal transients. The system, as shown in Fig. 5, consists of an offline circulation pump, a thermal-sleeved tee connection between the offline pump and the feedwater line, a connection line from the boiler downcomer to the pump, a warming bypass system, various valves, and a control system.
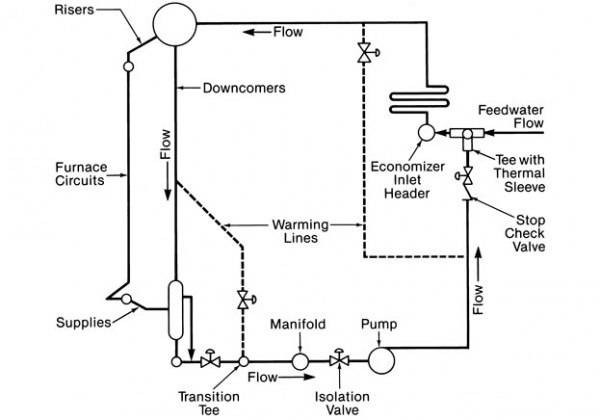
Fig. 5 Offline recirculation system to reduce thermal shock.
The offline pump is operated only when the boiler is shut down. Its purpose is to provide a small amount of circulation within the furnace circuit and through the economizer to prevent temperature stratification in the water circuits. The tee connection permits the introduction of a small amount of hot water from the furnace into the feedwater stream when feedwater is intermittently supplied to the boiler and before a steady feedwater flow is established. The warm furnace water introduced at the tee connection raises the feedwater temperature enough to prevent thermal shock to the economizer.
The connection contains an internal thermal sleeve that protects the tee from a thermal shock when cold feedwater is first fed to the economizer. A control system monitors the feedwater temperature and flow and controls the recirculation pump.
When the boiler startup sequence is initiated, the offline system is shut down and isolated. Warming lines permit natural circulation through the pump when it is shut down and the boiler is off line.
Experience with offline recirculation systems has shown that thermal shock differentials can be reduced to less than 100F (56C) from previous levels of 200 to 400F (111 to 222C). Such a reduction may eliminate the fatigue cracking that is associated with frequent unit cycling.
Load Cycling Effects and Solutions
The issues above are mainly related to on/off cycling. Load cycling to various ranges presents another set of unique challenges. The main challenges relate to maintaining stable combustion, and the ability to maintain air quality control system equipment (AQCS) in operation.
These challenges become more difficult to address as the desired minimum sustained load is reduced. Generally desired operating ranges can be classified as:
- turndown to higher values of approximately 40 to 50%,
- middle range of 25 to 40%, and
- the lowest range of house load (generating only enough electricity to power plant equipment) to 25%.
Flame stability
One of the major issues with load cycling is developing the necessary system(s) to maintain stable combustion during low load operation. Modern wall-fired burners provide well controlled air-fuel mixing. The amount of mixing varies with the firing rate of the burners. Acceptable air-fuel mixing is achieved at reduced boiler loads by operating with fewer burners in service and by operating those burners at higher firing rates. Burner mixing can be induced by using the primary air/pulverized coal stream, by using the secondary air, or by a combination of the two. Below a certain load the burners will not have the ability to maintain a stable flame without the use of auxiliary fuel for support.
To minimize auxiliary fuel costs during periods where flame stabilization is needed, duel capacity igniters can be utilized. The igniters operate at either of two capacities. One higher capacity will be at a class 1 input level at 10% of the coal burner capacity per NFPA 85. The other lower capacity would be at 5% of the coal burner capacity, which NFPA 85 defines as a class 2 igniter, the smallest permitted for coal ignition. When a pulverizer is being started the, igniters will operate at their full higher capacity, as required per NFPA 85. At all other times as permitted by NFPA 85, such as when igniter support is desired for extended low pulverizer loading, the igniter input can be reduced to the lower class 2 capacity. While class 2 igniters cannot be used in all situations that a class 1 igniter may be used, class 2 igniters are permitted to be used to stabilize the coal flame for low-load operation under specific prescribed conditions.
Pulverizer Turndown
Pulverizer turndown is a key in providing flexibility for boiler turndown. Generally, minimum turndown range is limited to two pulverizers in operation. A single pulverizer in operation with auxiliary fuel risks tripping the unit if the pulverizer trips. Primary air requirements are a function of the specific pulverizer design. Most pulverizers require 40 to 70% of their full-load primary air requirements at their minimum output level. In addition, the PA/PC mixture traveling to the burners must be transported at a minimum of 3000 ft/min (15 m/s). This velocity serves to prevent the coal particles from dropping out of suspension in horizontal runs of coal pipe. Minimum primary air flow is the greater of the minimum PA flow required for the pulverizer or the minimum required to satisfy burner line velocity limits.
The primary air and coal mixture conveyed to the burners reaches a maximum velocity and solids loading at full pulverizer load and follows the pulverizer output as mill loading is reduced. As the burner nozzle velocity increases, the ignition point gradually moves farther from the burner. At some point, continued increases in nozzle velocity can lead to blowoff of the flame, a potentially hazardous condition where coal ignition and flame stability are lost. The weight ratio of coal to primary air typically reaches a peak of 0.4 to 0.65 at full load and a minimum of 0.15 to 0.3 for minimum pulverizer load.
The combined use of the DSVS® classifier with spring load adjustor can improve pulverizer operating flexibility for reduced turndown. The tendency toward a courser product can be controlled by the classifier. The classifier can also be used to, in effect, increase the pulverizer working load at low outputs by increasing fineness to values higher than would be achieved with a static classifier. An increase in pulverizer working load at low outputs may simultaneously improve turndown and ignition stability.
If the economics of the low-load operation justify it, several options are available to provide uniform stable heat input. Natural gas firing provides uniform stable heat input to the furnace for operation down to and including house load. Dedicated smaller capacity pulverizers and burners are another option to provide the lower heat input from a lower cost fuel. Half mill firing is utilized to provide even further turndown with the existing pulverizers. In this design half of the burners are isolated while the other half operate in a more fuel rich environment providing stable minimum heat input. Excess fuel-laden primary air is routed to a baghouse where the fuel is separated and returned to the mill while the primary air is vented to the boiler. This excess primary air is metered to assure adequate combustion air is feeding the active burners.
Air Quality Control
Maintaining air quality control systems in service at lower loads presents a special challenge. Nitrogen oxides (NOx) can generally be controlled by combustion down to about 40% load with proper tuning and controls. NOx will increase as load drops from about 40 to 25%; in this case, the selective catalytic reduction (SCR) system goes out of service. Therefore, from a NOx control perspective, keeping the SCR in service to the lowest possible load requires one or more design solutions. To prevent the formation of ammonium bisulfate from forming in the catalyst, SCR inlet flue gas temperature should be maintained above a minimum injection temperature provided by the catalyst supplier. The following options can be used to maintain gas temperatures entering the SCR:
- Flue gas bypass – A portion of the flue gas is bypassed around the economizer and mixed with the flue gas that passes through the economizer.
- V-TempTM economizer – in this patented design the feedwater to the economizer is split into an overflow path and an underflow path, each feeding from its own economizer inlet header. The economizer tube bank consists of a mix of underflow and overflow tube sections fed from the underflow and overflow headers. Water through the overflow and underflow section is biased as load decreases and recombined in the mix headers.
- Split economizer – a portion of the economizer surface is relocated downstream of the SCR reactor.
- Water recirculation – a portion of water from a source near saturation, like a downcomer, is mixed with the feedwater to the economizer.
- Water bypass – a portion of feedwater to the economizer is bypassed around the economizer and mixed with the water that passes through the economizer.
- Surface removal – a portion of the convective surface (superheater, reheater, or economizer) is removed.
AQCS equipment located downstream of the air heater requires that the exit gas temperature remain above limits for proper operation as well. The equipment most susceptible to low gas temperatures at very low loads is a spray dryer absorber (SDA) and pulse jet fabric filter (PJFF). The recommended minimum average gas temperature entering this equipment is 220F (104C) for cold startup purposes. The minimum inlet gas temperature required to meet the specified SO2 emissions rate is a function of the inlet SO2 concentration and the atomizer feed slurry solids concentration. For a unit that is deep load cycled (house load to 25%), lower average gas temperatures may be possible based on testing and experience.
Boilers are designed to maintain the exit gas temperature of the air heater to control corrosion of the cold-end surface. The general assumption made is that the cold-end metal temperature is approximately equal to the average of the gas outlet and the air inlet temperatures. Most air heaters are designed to operate with gas outlet temperature above the acid dewpoint but minimum metal temperatures somewhat below the dewpoint, where the efficiency gain more than offsets the additional maintenance costs. The measures used to control the air heater exit gas temperature can involve heating of the entering air utilizing a heat exchanger, bypassing air around the air heater, hot air recirculation, or bypassing flue gas around the air heater. These methods have certain disadvantages that must be evaluated prior to implementation. The bypassing options, while more efficient at lower loads, suffer from undesirable leakage of air or gas around closed dampers at high load leading to efficiency loss. The most utilized solution of heating entering air via a heat exchanger may produce a higher heat rate penalty at the lowest load.